
Projects
Home > Projects
Research interests
Neural Circuits and Neuro-Glia Interaction Mechanisms Underlying Affective and Homeostatic Responses
Our daily lives expose us to various physiological and psychological stressors, and our ability to cope with these challenges is crucial to maintaining well-being. Inadequate coping with stress can lead to neurological and psychological disorders, such as anxiety, depression, and fear, as well as life-threatening conditions like dehydration. Several cortical and subcortical brain regions—such as the prefrontal cortex, lateral septum, hippocampus, hypothalamus, parabrachial nucleus, subfornical organ, and amygdala—are critical for affective and homeostatic responses.
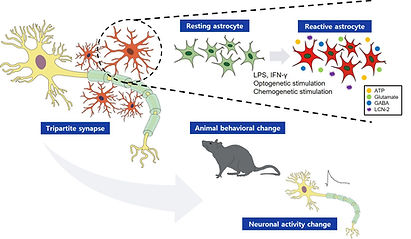
Recent advances in molecular genetics have helped identify molecularly and functionally distinct neuronal populations within these regions, revealing the functional heterogeneity among neurons. For instance, we previously identified specific neuronal populations mediating aggression and sexual behaviors in the hypothalamus. However, the mechanisms underlying emotional behaviors remain incomplete.
These brain regions also contain numerous glial cells, including astrocytes, microglia, and oligodendrocytes. While glial cells were traditionally thought to only provide homeostatic support to neurons, recent studies show that astrocytes actively interact with local neural circuits, playing a vital role in information processing and generating physiological and behavioral responses. Our research, along with others, has shown that astrocytes are involved in affective and homeostatic responses in these brain areas (see figure below). Still, the functional significance of most glial cells remains largely unexplored.
Therefore, our goal is to uncover the neuronal circuits that mediate emotional behaviors and homeostatic control, and their interactions with glial cells. We are using in vivo functional manipulation techniques, including optogenetics and chemogenetics, virus-mediated neural circuit tracing, brain slice electrophysiology, in vivo Ca²⁺ recording, anatomical and histological analysis, and behavioral assays.
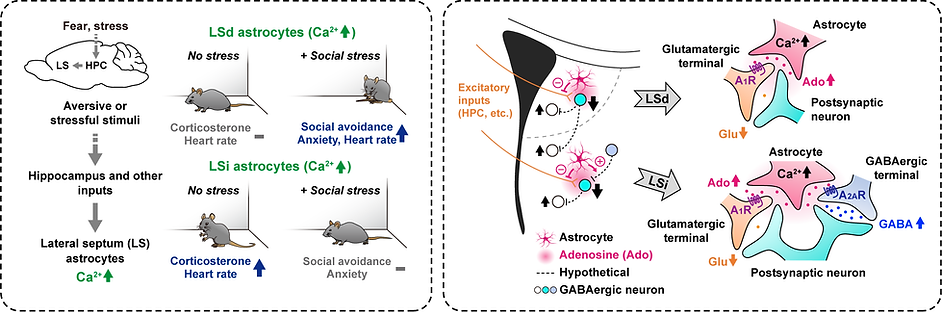
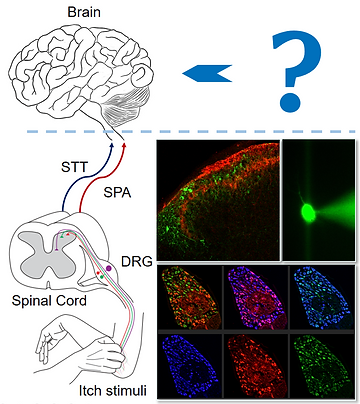
Molecular and Neural Circuit Mechanisms Underlying Somatic Sensation, Pain, and Itch
Pain and itch are unpleasant sensory experiences triggered by environmental and internal stimuli, tissue damage, irritation, diseases, or treatments like anti-cancer drugs. The somatic sensory system, which includes primary sensory neurons such as dorsal root ganglion (DRG) neurons, the spinal cord, and the brain, is responsible for the perception of pain and itch. Dysfunction in these tissues can lead to chronic pain and itch conditions.
The molecular and neural mechanisms underlying these sensations have been the focus of extensive research, aiming to understand how different sensory neurons, ion channels, receptors, and neural circuits contribute to their perception and modulation. Previously, we identified the roles of TRPV3 and TRPV4 in skin cells and sensory neurons using primary cultured cells, along with mouse knockout and transgenic models. We also studied the response kinetics of splicing variants of acid-sensing ion channels. Additionally, we clarified the functional distinctions between TRPV1+ and Mrgprd+ DRG neurons in somatic sensation and pain.
Currently, my lab is focusing on a specific type of potassium ion channel expressed in the DRG and spinal cord. We are investigating its tissue-specific roles in regulating DRG neuron activity and modulating neural circuits in the spinal cord. To achieve this, we employ mouse knockout and knockdown models, in vivo DRG Ca2+ imaging, electrophysiological recordings from acutely dissociated DRG neurons, and a range of behavioral assays related to somatic sensation, pain, and itch.
